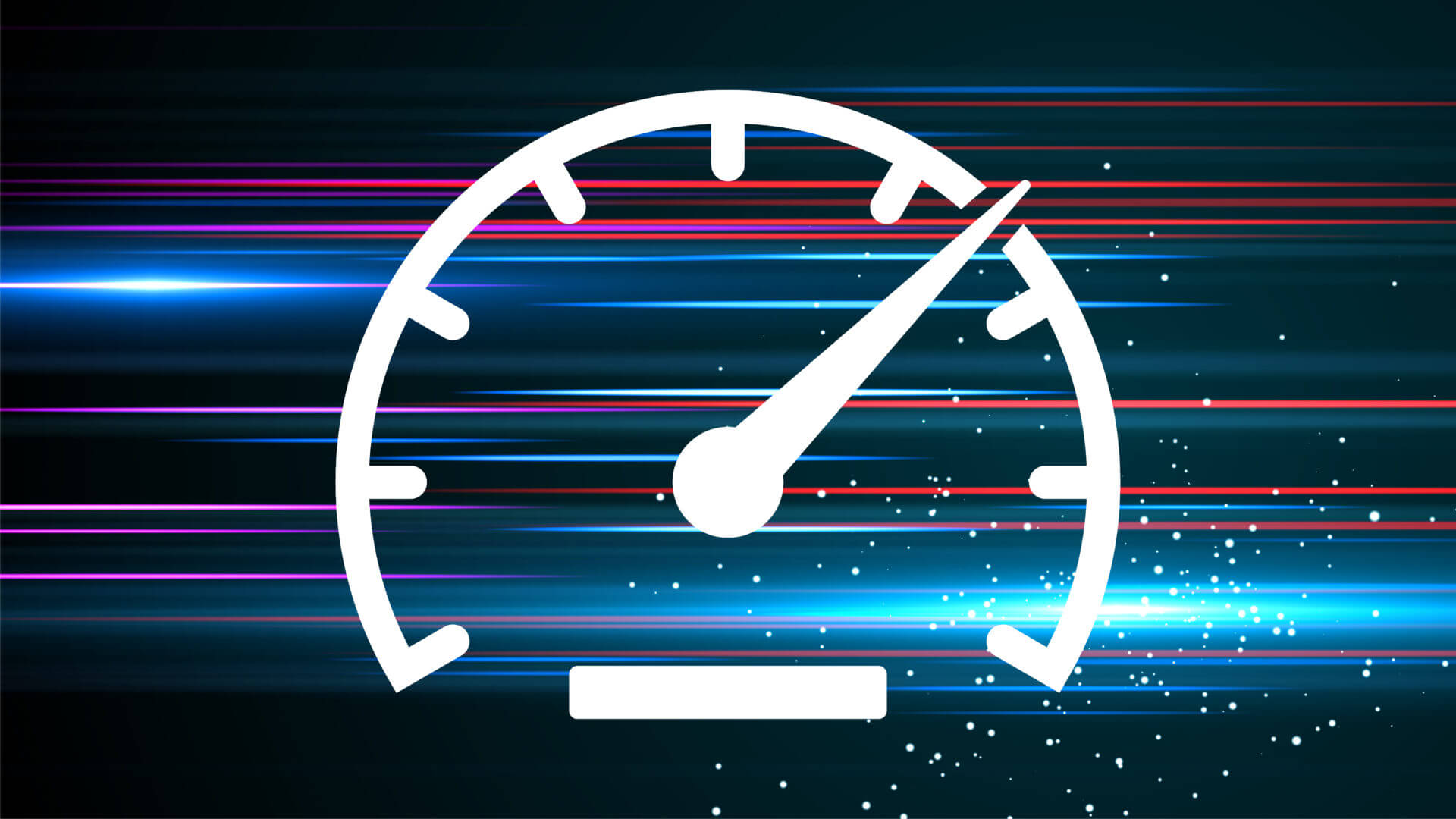
Optimizing VNA Measurement Speed
January 13, 2023Introduction
Vector Network Analyzer (VNA) measurement speeds are often estimated, and thus users strive to optimize their instrument’s measurement speed. Many are interested in the tradeoffs between speed, accuracy, and resolution in VNA measurements, especially when automating the instrument in a larger measurement system or production environment. This application note details the determining factors for measurement cycle time in Copper Mountain Technologies VNAs and optimizing VNA measurement speed.
Generally, a VNA measurement cycle consists of these components: A per-point tuning and settling time of the generator, a per-point measurement time, a per-sweep time needed to process and transfer results, and a per-sweep time for the generator to switch between output ports and to return to the start frequency. CMT instruments offer an additional user-programmable delay between points, which may be useful for integration with external equipment or DUTs with settling times of their own. Some of the delay components are effectively fixed, while others depend upon the measurement parameters and thus may be optimized by the user.
What Constitutes a VNA Measurement Cycle?
Based on the frequency range and number of points you set in the VNA software, the VNA calculates a list of frequencies that it needs to measure. A VNA measurement cycle is the process where the VNA measures all measurement points and transfers desired measurement data to the users’ program. The time it takes from the start of a VNA sweep to the end of the sweep is called sweep time. The time it takes for the VNA software to transfer data to the user’s program is called data transfer time. Cycle time is sweep time plus data transfer time.
When your VNA has more than 1 port, a port-switchover time may be added to sweep time based on what settings you have configured in the VNA software. This is because the VNA outputs power from one port at a time. Except for the TR series, all 2-port CMT VNAs have one source, and all 4-port CMT VNAs have two sources – Port 1 and Port 2 share one source, and Port 3 and Port 4 share the other source. Port-switchover happens when the VNA needs to switch the source to a different port. The port-switchover time is needed to wait for the source signal to stabilize after switching.
Fig 1. Timing Diagram for a VNA Measurement Cycle
What Constitutes the Measurement Cycle of Each Point?
A typical point measurement cycle looks like this:
- LOs programming – the time needed to load all the necessary data to the synthesizers. It depends on the number of registers to load, the speed of the communication interface (typically SPI), and some peculiarities of the synthesizers’ architecture.
- Transient time – the time required until all the synthesizers are stabilized, and the signal is spread all over the transmitting and receiving paths. Mostly depends on the synthesizers.
- ADC sampling – the time to get a window of samples that matches the selected IFBW. The formula is T=1.18/IFBW. For example, 100 kHz IFBW sampling time is about 12 µs (1.18/100 kHz).
- Calculating data – the time taken to calculate and prepare the data for transmitting it to the software. The typical time is 1.5 µs at 100kHz IFBW.
Generator Tuning and Settling Time
The first component of measurement time per point—the setup time—is determined by hardware and software limitations for generator re-tuning and settling. This time includes latencies associated with command and control internal to the VNA, as well as dependencies arising from the generator architecture such as settling times of an associated phase-locked loop (PLL) or voltage-controlled oscillator (VCO) necessary to achieve a stable output signal frequency and power level for the next measurement point. Setup Time depends on frequency; however, the step size can quickly become difficult to model and therefore is not fully characterized in a VNA’s performance specification. Instead, typical cycle measurement times are provided for the VNA for a particular start and stop frequency and number of points. Usually, at least one such frequency range will be the full span of the instrument, which provides something of an upper bound on the setup time, as the time is generally lower for smaller frequency spans. The setup time cannot be optimized by the user in general, since it is constant for the hardware of a given VNA. However, the Setup Time does often vary depending upon the frequency and inter-frequency step size, therefore the stimulus frequency range or point density is flexible in your measurements. This can be used to optimize measurement time.
Measurement Time
The second component of the measurement time per point—the measurement time—includes a variable aperture time necessary for measurement that is based on the settling time for the IF filter. Narrower IF filters require more time to ring out or settle. The measurement time in seconds is generally equivalent to an IF Bandwidth (IFBW) coefficient divided by the user-selectable IFBW in Hz. Copper Mountain Technologies Full Size, S, TR, R, and Cobalt series VNAs have an IFBW coefficient of 1.18; depending on the max IFBW of the VNA, one can calculate the minimum per-point measurement time.
The actual Measurement Time of the instrument is slightly longer than the IFBW settling time, as it also incorporates fixed delays associated with digitization and internal data transfers. These fixed delays are negligible compared with the IFBW delay and are significant to a certain extent, thus they can be considered as part of the Setup Time.
There is a tradeoff between IFBW/ Measurement Time and Dynamic Range. The figure below illustrates the effects of using a wider IFBW on Settling time and Dynamic Range for the SC5090 VNA, a 2-port instrument that measures from 300 kHz to 9.0 GHz. The IFBW can range from 1 Hz to 1 MHz and the dynamic range at 1 Hz is 150 dB.
Fig 2. Dynamic Range, IFBW Settling Time vs IFBW
Since the IFBW is the dominant variable component of the per-point measurement speed of the VNA, its selection is crucial when trying to optimize measurement speed. Widening the IFBW will decrease measurement time per point, but also decrease the dynamic range of your measurement. For every doubling of the IF Bandwidth, the dynamic range decreases by 3 dB, so you lose 10 dB of dynamic range per decade. The IFBW coefficient is 1.18, so the selection of an IFBW between 1 Hz and 1 MHz corresponds to a settling time between 1.18 sec and 1.18 µsec.
If the dynamic range of an instrument is specified to be 150 dB at 1 Hz IFBW, then at 1 MHz IFBW, the dynamic range will be 90 dB according to the below formula:
150 dB – 10log10(1 MHz /1 Hz) ≈ 150 dB – 60 dB = 90 dB
Return Time
Beyond the per-point delays, the full measurement cycle for the instrument also includes fixed and variable delay components. The fixed components include a port switchover time to change the generator between ports, a fixed time for returning the generator to its start frequency, and a small USB latency between sweeps.
While the port switching time is a fixed delay, users can specify the number of sweeps and which parameters are measured, and by so doing can perhaps minimize the number of port changeovers. When a port changeover is not required between sweeps, the delay is much smaller and may be termed Return Time. In Copper Mountain Technologies VNAs, the Return Time is the sum of sweep frequency return time and USB latency, and is typically several milliseconds, though this time will vary based on your PC, the USB controller, and how many USB devices are connected to the USB controller.
How to Estimate Cycle Time
Cycle time can be estimated based on the Measurement Time per Point spec for each instrument shown in the table below. The measurement time per point in this table is with the maximum IFBW allowed for each instrument and is measured by having a lot of points and dividing the total cycle time by the number of points. Thus, using this table to estimate cycle time is more accurate when estimating for more points. This is because the computer’s overhead has more impact when the measurement has fewer points. Table 1 shows the measurement time per point for a list of CMT VNA models.
Table 1. VNA Measurement Time Per Point, Dynamic Range, and Effective Directivity Specs
How to Measure Cycle Time
To measure cycle time when the measurement is not automated by a program, you can use our VNA software’s built-in Cycle Time feature. To enable it, go to Display> Properties>Cycle Time and turn it on. This feature measures cycle time by starting the timer right after the end of the previous sweep. This means this feature measures cycle time accurately only when you are using VNA’s internal trigger (which will be the case when the measurement is not automated). If you are using an external or BUS trigger, the cycle time measured by this feature will include the delay between triggers and this will make the cycle time measurement inaccurate. Because of this, when you automate the VNA and trigger the VNA by BUS or external sources, you can’t use this built-in feature for accurate cycle time measurement.
To measure cycle time when using external or BUS trigger, use timers in your program. Some examples of this can be found under SCPI/Python and SCPI/C++ folder in our programming examples, which can be downloaded here:
https://coppermountaintech.com/programming-examples/
Steps for Increasing VNA Measurement Speed
A VNA with better performance
Different VNA models have different hardware, so they have different measurement speeds even if you use the same stimulus settings (number of points and IF Bandwidth). Besides these differences, different VNA models also have different port-switchover times.
Higher IF Bandwidth (IFBW)
Narrowing the IF bandwidth reduces the noise and widens the dynamic range but the measurement time increases. Increasing the IF bandwidth gives a better measurement speed but compromises the dynamic range. This difference in speed is due to the different speeds of LOs. Use the widest IF Bandwidth that is acceptable for your measurements.
Lower Number of Points
Use a lower number of points for faster measurement speed and a higher number of points for better resolution in frequency. There is a tradeoff between speed and frequency resolution.
Lower Number of Channels
Use as few channels as possible. All the channels sweep sequentially, thus having unnecessary channels will slow down your measurement.
Only Display Necessary Traces
Only have the traces necessary for the desired measurement. For example, if you only want S11 measurements, you should not have a trace measuring S22, as the VNA will switch the source to Port 2 to make unnecessary S22. Having fewer traces may not increase measurement speed, because calibration also plays a major role in measurement speed. For example, the full 2-port calibration algorithm requires the measurement of all four 2-port S parameters. When such a calibration is active, even if you only have a trace to measure S11, the VNA still will switch source to Port 2 to measure S12 and S22.
Choose the Right Type of Calibration
Perform only the type of calibration that your measurements require. For example, if you want to see the response of all four S parameters of a two-port VNA, perform 2-port calibration, if you only want to see an S11 response, perform a 1-port calibration on Port 1.
Use Segmented Sweep
The sweep can be divided into segments and the stimulus settings can be adjusted for each segment. You can skip less important frequency ranges or have higher IFBW settings for these ranges to increase measurement speed.
Use Invisible Mode
Launching the VNA software in Invisible Mode will hide the VNA software UI and will provide a faster and more stable measurement speed, as the VNA software does not spend time rendering graphs to update the display. There are two ways to turn on Invisible Mode:
- Launch through Command Prompt (cmd.exe):
For S2VNA and S4VNA:
C:\VNA\S2VNA\S2VNA.exe /SocketServer:on /SocketPort:5025 /visible:off
For RVNA and TRVNA:
C:\VNA\RVNA\RVNA.exe EnableSocket:5025 InvisibleMode
- Turn on/off Invisible Mode using SCPI commands:
Send “SYST:HIDE” to turn on Invisible Mode and send “SYST:SHOW” to turn it off.
Turn Off Display Update
Turning Display Update off will provide a faster and more stable measurement speed for the same reason as turning on invisible mode. Having Display Update off means the traces will not update. The software UI will not be hidden, as opposed to when the Invisible Mode is turned on.
Table 3. Cycle Time Comparison for Display Update On and Off
Use Narrower Frequency Span in Stimulus Settings
The VNA internally switches paths when measuring different frequency ranges. When switching happens, the VNA will use a time delay to wait for the signal to stabilize. This adds to the cycle time. Table 4 shows a comparison between two extreme cases of frequency span – full span and zero span.
Table 4. Cycle Time Comparison for Zero Span and Full Span Frequency Stimulus Settings
How to Increase Data Transfer Speed
Use Binary Data Transfer
When automating the VNA using another program, one extra step is added to the measurement cycle. After the VNA finishes a sweep, you should transfer the measured data to your program so that it can be post-processed. Data transfer time is negligible because it is very short, especially when compared to the cycle time of measurement. If you are automating the VNA using SCPI commands through TCP/IP Socket or HiSLIP connection, the data transfer is done by default by sending ASCII characters. In this case, 17 characters are used to represent each number. Each character is 1 byte, so each number is 17 bytes long. If the VNA is configured to transfer data in 32-bit binary format, 4 bytes will be used to represent each number. Because the VNA is now sending 13 bytes less for each number, the data transfer speed will be increased. CMT VNAs support both 32-bit binary format and 64-bit binary format. To enable binary data transfer, use the SCPI command “FORM:DATA <format>” to select data transfer format and “FORM:BORD <endianness>” for the endianness of the binary data. We have several examples on binary data transfer in Python in our programming examples. They are inside the SCPI(recommended)/Python folder. Our programming examples can be downloaded here:
https://coppermountaintech.com/programming-examples/
Table 5. Data Transfer Time Comparison for ASCII, REAL64, REAL32 Data Formats
Use Hislip Instead of Socket Connection
Hislip protocol is faster than Socket protocol, although they are both TCP/IP based. The advantage of Hislip is only more apparent when the number of points is larger. Table 6 shows experimental data on data transfer time using Hislip and socket.
Table 6. Data Transfer Time Comparison for Hislip and Socket Connections
Conclusion
The total measurement cycle time for a VNA consists of fixed delays as well as several parameters which are under the user’s control. Accurate estimation of the cycle time for a given set of stimulus and measurement parameters is straightforward once the delays involved are well-understood. Software timers must be used to measure cycle time.
There are many ways to increase measurement speed and data transfer speed for CMT VNAs. Using binary data transfer can greatly reduce data transfer speed with a large number of points.