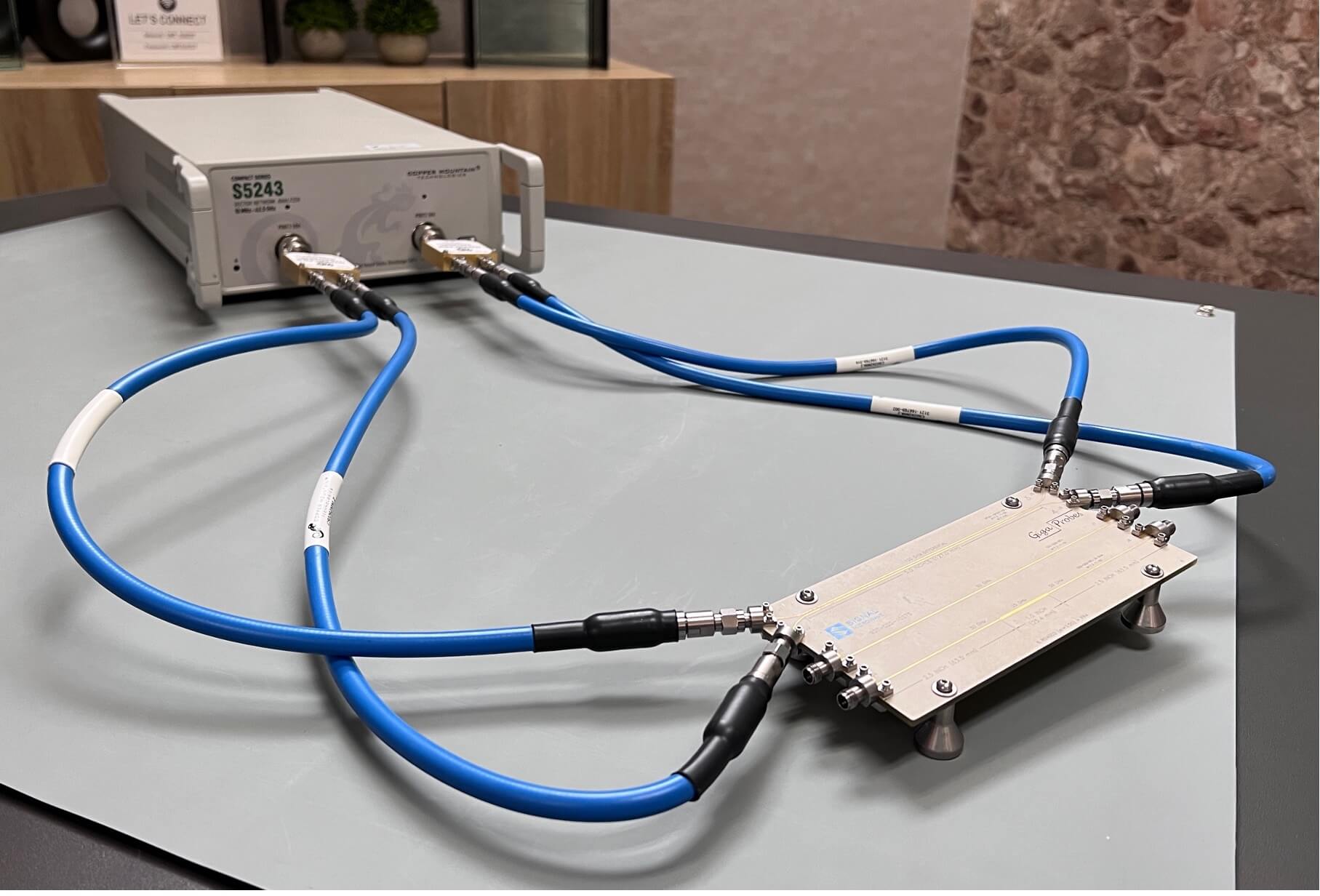
Making High-Frequency Balun Measurements
May 1, 2023Introduction
Balanced measurements to very high frequencies are becoming more and more commonplace. Twisted pair cables are expected to pass digital data with frequency content as high as 18 GHz or more. SRDES lanes on printed circuit boards may require bandwidths just as high. High speed differential amplifiers are needed to drive ADCs and must be properly evaluated. It is possible to compute balanced differential measurements from a series of unbalanced measurements for passive media, such as cables, but this requires a four port VNA. Differential amplifiers require a real differential drive that can only be provided by a balun, as shown in Figure 1.
Figure 1 – Differential Amplifier
Fully understanding how to implement a balun measurement is critical to achieving acceptable results.
How Does a Balun Work?
Theoretically, a balun should take in a single-ended, unbalanced signal and generate a balanced signal with 180° phase difference between the outputs. It should also work in the reverse sense to convert balanced into unbalanced signals, hence the name balun. If the two legs of the balun output are not precisely 180° out of phase, you can model the output as a balanced signal plus a small common mode signal. A less than perfect balun with an unbalanced input on its input port will always create a balanced signal with a small amount of common mode signal.
When used in the opposite direction—that is, converting a balanced to an unbalanced signal—any common mode (signals in phase) present on the two inputs should not influence the unbalanced output in any way. A less than perfect balun will always exhibit a small amount of this error. Figure 2 shows the common mode errors in and out.
Figure 2 – Balun Errors
When Common Mode signals are created on the balanced output port due to the single ended input, this is called mode conversion. Reflections from the balanced port may also exhibit mode conversion. Theoretically, if the single-ended port is terminated with 50 ohms, a balanced signal incident on the balanced port should be mostly absorbed, with a small balanced reflection. Some of the balanced incident signal may also create a small mode converted common mode reflection. An incident common mode signal should create a large common mode reflection but will also create a small mode converted balanced reflection. The two mode conversion reflections are shown in Figure 3 below.
Figure 3 – Reflection Mode Conversions
What are Mixed Mode Parameters?
We can define all properties of a Balun using mixed mode parameters. These are:
- SSS11 is the return loss looking into the single-ended Port 1.
- SSD12 is the single-ended signal developed on Port 1 from the balanced signal on Port 2.
- SSC12 is the single-ended signal developed on Port 1 by the Common mode signal on Port 2. This should be a very small number for a good balun.
- SDS21 is the differential thru response of the balun.
- SDD22 is the differential Port 2 return loss.
- SDC22 is the differential mode reflection from Port 2 due to a Common mode signal exciting it.
- SCS21 is the Common mode signal generated on Port 2 by the single-ended Port 1 input. This should be very small for a good balun.
- SCD22 is the Common mode reflection from Port 2 due to a differential excitation to it, small for a good balun.
- SCC22 is the Common mode reflection from Port 2 due to a Common mode excitation to it, should be large. Common mode should be mostly reflected.
Two additional ratios are often used:
- CMRR1 = SDS21/SCS21, the ratio of the differential to Common mode signal generated on Port 2 by a signal on Port 1. A large number is good.
- CMRR2 = SSD12/SSC12, the ratio of the single-ended signal generated on Port 1 by a differential signal on Port 2 to the one created by a single-ended signal on Port 2. A large number is good.
Each of these parameters define either a desired or undesired property of a balun, and the last two ratios provide a figure of merit.
It is common for SDD22—the differential return loss—to be fairly poor on many baluns, perhaps 10 dB. If this is the case, add a 6 to 10 dB pad on each output to improve the return loss by 12 to 20 dB. This will reduce reflections and flatten the frequency response of the balun.
How Do These Signals Combine for a Balun?
If the balanced side of the balun is broken into Common Mode and balanced ports, the signal flow graph may be drawn as shown in Figure 4. The red arrows show mode conversions taking place. The C and D ports on the right-side feed Common Mode and Differential Mode signals to the next stage of the system. If that next stage were a differential amplifier, it would undoubtedly exhibit some amount of mode conversion and the Common Mode input signal would create a small differential output along with the main differential to differential response. This creates ripple in the amplitude response.
Figure 4 – Mixed Mode Balun Parameters
For example, if the Common Mode signal on the output of the amplifier is 20 dB down from the desired differential signal, there would be approximately 1 dB of ripple on the output signal. This estimate comes from the addition of two signals stated in log terms:
What Does the System Response Look Like?
If a pair of baluns is used to measure a balanced device, the desired and undesired signals—ignoring reflections— may look like the block diagram below:
Figure 5 – Balanced Device Measurement with Errors
The signal path in black is the desired, error-free measurement, where the dashed red lines show contributions from mode conversion, which cause undesired ripples in the final amplitude response.
Example Measurement
A 100Ω differential line on a PCB was measured using a 20 GHz 4-Port VNA displaying the calculated differential properties and then measured again using a pair of high frequency baluns.
Figure 6 – Differential Line Measurement
The test board in Figure 6 was provided by Signal Microwave/Giga Probes. This high frequency test board has a known performance response to 40 GHz and can be used to verify VNA calibrations and check for measurement drift. They are also teaching tools for VNA users. This board has a 100Ω differential line, a 50Ω line, and a 50Ω line with a short 25Ω section for demonstration of time domain analysis.
Figure 7 – Differential Measurement with 4-Port VNA
The same differential pair was measured using a 40 GHz 2-Port VNA and 40 GHz baluns from Hyperlabs.
Figure 8 – Measurement Using Baluns
Figure 9 – Differential Line measurement with Baluns
The results show very good agreement. Note that the 4-Port measurement stopped at 20 GHz and the 2-Port measurement with baluns was made to 40 GHz. In Figure 9, a small amount of ripple is seen in S21 and S12 at the very highest frequencies. This might be improved using phase adjusters on the lines. The specifications on the Hyperlabs baluns are quite good and the results were excellent. The cables shown in Figure 8 were chosen for best delay and phase match.
How are Mixed-Mode Parameters Calculated?
A full 3-port measurement of a balun can be used to determine all of the mixed mode parameters. If Port 1 is the single ended port and Ports 2 and 3 are the differential port, then:
The 4-port UI software from Copper Mountain Technologies will perform all of these calculations on a 3-port Touchstone file automatically and can be downloaded here. Be sure to look through all of the Vector Network Analyzer products on our website.
References
[1] Brian Walker, “Online Spotlight: Balun Measurements with a Two-Port Vector Network Analyzer”, Microwave Journal, July 14, 2022
[2] K. Suto and A. Matsui, “Two-Port S-Parameter Measurement of Wide-Band Balun,” Proceedings of the International Symposium on Antennas and Propagation, October 2016.
[3] R. W. Lewallen (W7EL), “Baluns: What They Do and How They Do It,” ARRL Antenna Compendium, Vol. 1, 1995, pp. 157-164, Web: www.eznec.com/Amateur/Articles/Baluns.pdf
[4] “Measurement Techniques for Baluns,” Anaren, May 2005, Web: https://shop.richardsonrfpd.com/docs/rfpd/Anaren_De-embed-BalunTesting_applicationnote.pdf