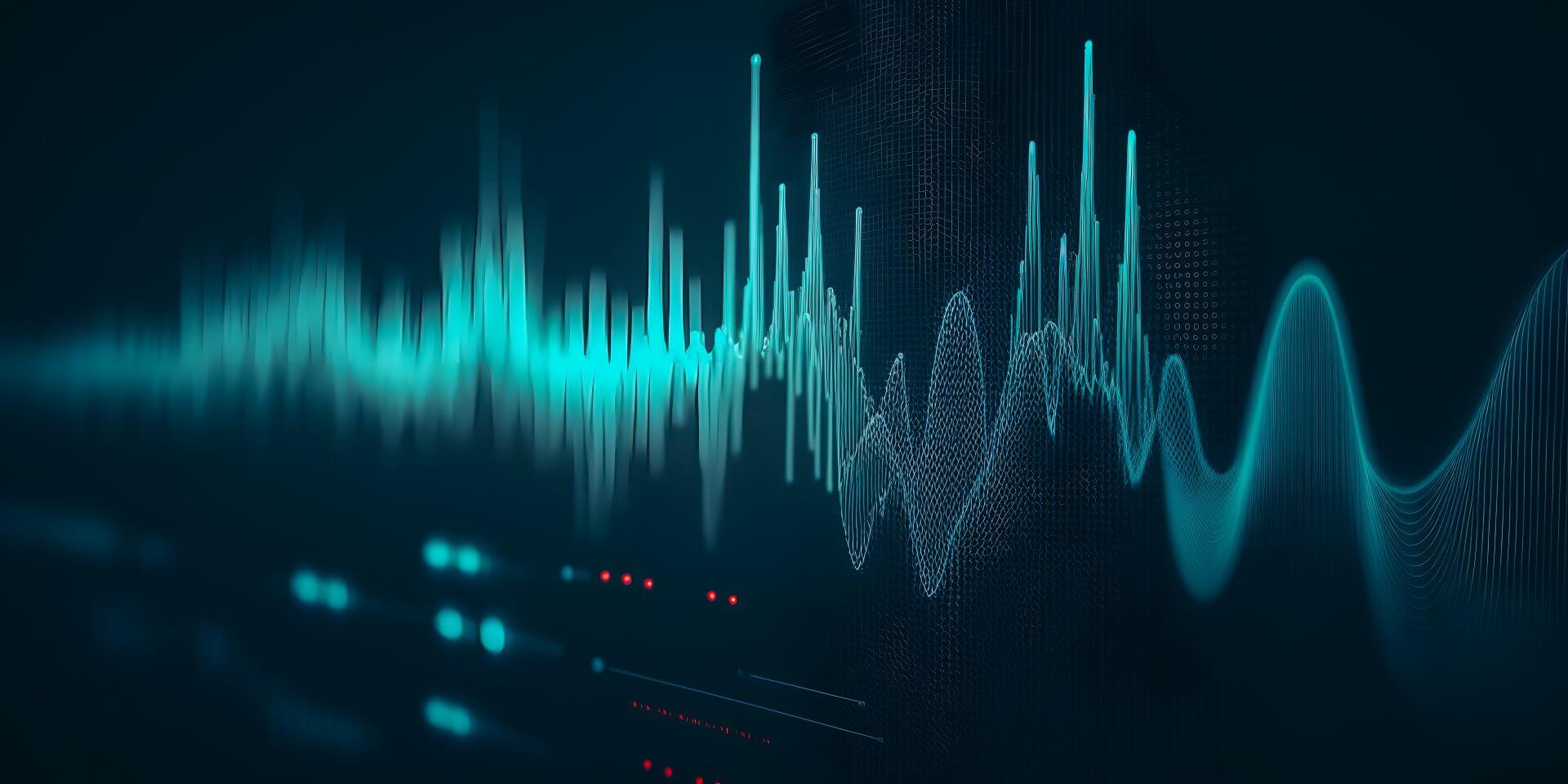
VNA Gating with Time Domain Processing
July 18, 2024Introduction
A Vector Network Analyzer (VNA) is typically used to characterize devices such as filters, antennas, amplifiers and other RF devices. The VNA makes S-Parameter reflection measurements. These measurements are sufficient to completely model the performance of any linear RF device. For instance, a Touchstone file containing a table of measurements may be dropped into a linear simulator program such as AWR as a component. The designer can then predict the performance of the device with various source and load impedances and at different frequencies. In addition, VNA Gating with Time Domain Reflectometry or TDR measurement with a VNA is possible.
In the past, a very sharp and narrow pulse was sent down a cable and any reflections could be evaluated to determine the health of the cable. A narrow, sharp pulse is equivalent to emitting all frequencies at equal amplitude. A VNA can create these component frequencies one at a time, and measure each reflection, accomplishing the same result. Inverting the results with an Inverse Fourier Transform results in a measurement of Reflection vs Time along the cable so that specific impairments can be picked out. With the VNA gating function, chosen impairments in the Time Domain can be “zeroed out” using a window function and then after transforming back to the Frequency Domain with a Fourier Transform, the response of the cable without the impairment can be evaluated. Also, a window function might be applied to the Time Domain response to pick out a particular section for analysis and ignore the rest.
Let’s start with the basics first.
What is a VNA?
A VNA is an RF test instrument which generates RF signals which can be sent through a Device Under Test (DUT) and measure signals reflected back toward the source. Each port of the VNA has a Bridge which separates signals leaving the port from those entering the port. A 2-Port Vector Network Analyzer block diagram is shown below in Figure 1. The Stimulus signal passes through either the Port 1 or Port 2 bridge and leaves the VNA. A sample of the exiting stimulus signal appears on the R1 channel, perhaps 20 dB down. Similarly, if a signal enters Port 1, it appears on the A channel. The R2 and B channels on Port 2 perform similarly. An LO signal tracks the Stimulus frequency with a constant offset.
The four channels are routed to four mixers and the IF outputs are a constant frequency determined by the difference between the stimulus and the LO.
Figure 1 – VNA Block Diagram
The four IF signals are digitized—although only one of the R channels are active at a time—and passed to the internal FPGA. The FPGA mixes the IF signal down to zero with a Numerically Controlled Oscillator (NCO) and the resulting I and Q signals are routed to DSP Finite Impulse Response (FIR) filters to apply the desired IF bandwidth. The now reduced bandwidth data is heavily decimated—keeping perhaps one of each thousand points—and the now highly reduced data rate is piped over the USB bus to the host computer for application of calibration and final processing by the VNA software.
Vector Network Analyzer Basics, S-Parameters
The VNA emits a signal which can be characterized as a voltage wave traveling away from the emitting port, the incident wave V1, shown in Figure 2. If the input of the DUT is not perfectly matched, not 50 ohms, there will be a reflection, V2 from it which travels back to Port 1 of the VNA. The remaining signal which is not otherwise consumed by or radiated away from the DUT is passed on to Port 2 as V3. S-Parameters are simply the ratios of these voltages. S11 would be V2/V1 and S21 would be V3/V1. The subscripts of S21 mean the voltage seen at Port 2 with Port 1 providing the stimulus. S11 means the signal received by Port 1 with it also providing the stimulus. In other words, a reflection.
Figure 2 – Incident and Reflected Voltage Waves
When Port 2 is providing a stimulus, we will have S22 and S12. The voltage reflected back to Port 2 and the voltage passed to Port 1 with Port 2 providing the stimulus, respectively.
What is the difference between S11 and S21 in a VNA measurement? S11 is a reflection measurement and S21 is a transmission measurement. S11 measurements are often called Return Loss (RL) and S21 Insertion Loss (IL). These terms are very often used when filters are being measured.
What Can you Do with a Vector Network Analyzer?
A Vector Network Analyzer has many uses in RF Engineering. A receiver will have an RF input section with amplifiers and filters. The gain of the amplifiers and insertion loss and rejection of the filter can be analyzed with a VNA. The RF section will generally lead to a mixer which converts the high input RF frequency to a lower Intermediate Frequency (IF). The Conversion Loss of the mixer can be measured using a VNA with a Frequency Offset mode—the stimulus signal leaving the VNA at the RF frequency and the receiver on the other VNA port set to the IF frequency. The effectiveness of the antenna feeding the receiver may be verified by measuring its Return Loss—the relative amount of signal reflected back to the source vs that which is radiated. An antenna is a reciprocal device, so it is acceptable to measure its radiation efficiency to evaluate its effectiveness for receiving signals.
An Engineer designs RF circuits based on the data sheet specifications of the components, but the actual performance must be verified in a prototype circuit and a VNA is the ideal tool for this.
What is the Time Domain Option in a VNA?
What does TDR mean in transmission line analysis? TDR stands for Time Domain Reflectometry and was once performed by sending a voltage impulse into a transmission line in order to see the reflections caused by impairments somewhere along the cable system, much like a radar. As mentioned previously, we can mimic the effect of an impulse, a delta function, by sending out its components one at a time. Differing frequencies are mathematically orthogonal and when we are dealing with a linear system, the principle of superposition applies. We can analyze the linear system with a true impulse or with a series of component frequencies one at a time. We use this to phenomenon to perform time domain analysis using a Vector Network Analyzer.
The old-school voltage impulse method required a wide-band oscilloscope to view the resulting reflections, perhaps as wide as a GHz or more. A VNA measuring one frequency component at a time can perform each measurement in a low bandwidth, as low as 10 Hz. The noise power difference would be 80 dB which spectacularly improves the dynamic range of the VNA Time Domain measurement compared to a voltage impulse measurement.
In the Time Domain Analysis mode, we sweep a transmission line from a Start frequency to a Stop frequency in small frequency steps. With the stimulus set to Port 1 and measuring S11 we will see any reflections which occur along the cable length or at its end where it will be connected to some device. The connector at the end will certainly reflect some energy, as will the connected device unless it is very well matched to the characteristic impedance of the cable. The reflection data at each frequency is recorded and an inversion is performed to get to the Time Domain. We might use an Inverse Fourier Transform to accomplish this. If graphed, the result shows the magnitude of reflection at all frequencies vs the time for the signal to leave the VNA, impinge on a reflector and return.
In practice, an Inverse Chirp-Z Transform is used to produce the Time Domain response as it is more versatile than the Inverse Fourier Transform, having the ability to “zoom in” on any given section of the Time Domain result.
It is a simple matter to divide the time scale by two, since we are interested in the time to get to the reflection, and we can change the scale to distance by multiplying time by the velocity of the wave in the cable, or guide velocity. The guide velocity may also be expressed as the free-space velocity multiplied by a velocity factor. The free-space velocity is c, or 3*108 m/s and the velocity factor is material dependent, about 0.65 for solid PTFE dielectric cables.
The Time Domain calculation uses the reflection coefficients at all frequencies in the sweep, so it isn’t possible to draw any conclusions about reflections at any one frequency or those in a narrow band within the sweep. The resulting time step in the Time Domain plot will be the inverse of the sweep bandwidth, 1/(Stop-Start). The total time will simply be the number of points in the sweep multiplied by the time step. The greater the number of points, the longer the total time and the greater the distance which might be analyzed. Potentially, for a very low loss medium, it is possible to analyze thousands of feet. A larger sweep bandwidth equates to a smaller time step and hence, finer resolution in the plot. An 18 GHz wide sweep would have a 55 pS time step. 55 pS equates to about 0.42” in PTFE cable. If we use 10,000 points that’s a 352 foot range, enough to analyze the health of an RF cable leading from a base station to the top of a radio tower.
As an example, let’s look at two cables connected with an N-to-N adapter.
Figure 3 – Time Domain Cable test
With a 3.5 mm calibration Load applied to the end of the two cables, the reflection vs frequency or Return Loss is quite good, better than 25 dB to 6 GHz as seen in Figure 4.
Figure 4 – RL of Two Joined Cables
Now enabling Time Domain analysis, we can analyze the sources of reflections in the cable assembly. Figure 5 shows this. The adapter in the center is pretty good, being no worse than the cable connectors and there are no impairments along the cables themselves. Each of the 7 major sources of reflection in the cable assembly reflections back to the source which constructively or destructively interfere, causing the ripples in the response of Figure 4. If it weren’t for the connector reflections, the overall return loss of the cables would be phenomenal.
Figure 5 – Time Domain Analysis of Cable Assembly
Finally, let’s investigate Time Domain Gating.
What is Time Domain Gating?
In the Time Domain, we can isolate reflections and because of this, we can choose to zero out particular reflections or pick them out to look at them separately. After doing this, we can perform a normal Fourier Transform to return to the Frequency Domain to evaluate the results of our modification. To remove a reflection, we zero it out gently with an inverted window function. Simply squashing a section to zero would result in discontinuities and create “ringing” artifacts in the transform. Similarly, to focus on a particular reflection area we can multiply the Time Domain data by a positive window function. Positive and negative windows are shown below in Figure 6. (The X axis is arbitrary)
Figure 6 – Hamming Windows
We can use a negative window on the Time Domain response of Figure 5 to completely remove the reflections from the adapter as shown below in Figure 7.
Figure 7 – Notched out Adapter Reflections
With the reflections from the adapter removed, the overall return loss of the cable assembly improves by about 5 dB.
Figure 8 – Improved Return Loss with Adapter Reflections Removed
If a DUT is mounted on a circuit board with connectors and traces, a positive gate may be placed at the DUT to eliminate all other reflections and effectively de-embed it. This method is not perfect as any large reflections ahead of the DUT reduce the signal level which reach it. This artificially improves the return loss of the DUT.
Conclusion
We’ve learned the basics of VNA measurement and seen how the results can be post-processed in the Time Domain and how Gating may be used to eliminate or focus on certain reflections. Time Domain and Gating analytical functions come as standard features on all Copper Mountain Technologies VNAs except for the value leader “M” series. All our VNAs exhibit world-class performance and deliver metrology grade results traceable to the National Institute of Standards and Technology. See these great products on our website, www.coppermountaintech.com and feel free to peruse our vast library of technical articles, videos and webinars.